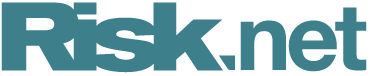
Power surge: the value of investing in renewables
Energy market expert investigates ways to forecast future power prices and capture rates in order to value renewables PPAs
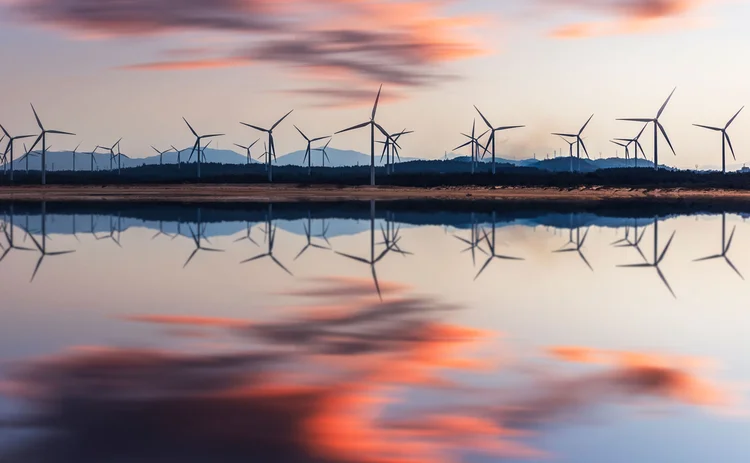
Valuing an investment in renewable power requires a full understanding of future power prices. In fact, if the asset is a merchant operation with no feed-in tariff, the power price is the main value driver. Price is also the main risk factor in a power purchase agreement (PPA) contract.
Therefore, understanding the methodologies available to forecast electricity prices over the short- and long-term is key to negotiating a PPA or valuing an asset. While traded power markets can certainly provide some shorter-term data, this has to be supplemented with fundamental analysis. Even in the most liquid power markets such as Germany, Italy and France, where a range of contracts are traded on exchange and over-the-counter, liquidity is scant for time horizons over three to five years.
Moreover, baseload calendar forward prices are not sufficient to assess the value of a renewable asset or PPA contract. A typical generation profile is not baseload and is not constant over the year. Eventually, a forecast with hourly granularity is required. An hourly price forward curve in power markets is essentially a mix between market and model, sometimes with more market and sometimes with more model depending on the availability of accurate market data.
Two main patterns can be distinguished: seasonal (monthly) and within-season (daily and hourly). Both types of price patterns are dependent on the dynamics of demand and renewable electricity production. For example, markets with large solar capacities tend to have a low price level in the summer in the middle of the day when sunshine is abundant. This could be partly offset by extra demand for air conditioning. In the same way, wind generation is often more abundant in winter, reducing price levels, but countries with a lot of electric heating or heat pumps have high electricity demand in winter.
Seasonal discrepancies between countries and regions can be huge. An interesting comparison is between Germany and the Oslo area in Norway, part of Nordpool (see figure 1). In Norway, the dominant renewable generation is hydro, with a production share close to 100%. Due to the melting of snow and ice, there is more water in the spring and summer than in the winter and hence lower summer than winter prices. But apart from that, there is little variation in the prices: there is abundant flexibility to ramp up or down the production at virtually no marginal cost.
Compared to Germany, where coal-, lignite- and gas-fired plants are the dominant marginal assets, Norway has a very flat hourly pattern, and a level almost always below Germany.
Building forward curves
The forward curve in figure 1 is derived from current and historical power market prices only. Based on this limited set of market information, the goal is to construct a forward curve with hourly granularity. In some markets, such as the UK and Japan, half-hourly granularity would be required. This curve should:
- be arbitrage-free to the market prices, meaning the average of the hourly prices in a certain period (for example 2022) should be exactly equal to the traded price for that period (for example, baseload 2022 trading at €35 per megawatt hour (MWh); and
- have the correct seasonal, weekly and hourly shapes. This means the shapes should reflect the ‘expected’ patterns in future spot markets.
An example of the forward curve-building process is given in figure 2, which shows data for the Spanish power market on May 8, 2020. Spanish power futures are traded on the European Energy Exchange (EEX) for individual months, quarters and calendar years.
The vast majority of liquidity is in the shortest maturities, mainly month-ahead and year-ahead. For the curve-building, we took settlement prices of the first three months, three-quarters and three calendar years ahead. The first step of the curve-building process is to derive monthly forward prices. The first three months (June, July and August 2020) are the settlement prices. The next month, September 2020 is easily derived to be €34.63 by taking the quarter-ahead (July to September 2020) and the July and August prices: the hourly weighted average of the three months should equal the quarter-ahead price.
While this works in liquid products, in lower liquidity products there can be a potentially unrealistic shape produced in the curve. Therefore, choosing a tradable horizon is important for each type of product.
In the example in figure 2, from October 2020 onwards, the information is much more limited. The two remaining quarter-ahead prices and year-ahead prices must be broken down into months. This requires shaping factors that are derived from historical forward price relationships.
For example, if the February forward price has historically been trading around 12.5% above the March forward price, then it is reasonable to enforce this relationship on the February and March prices in 2021 to 2023. Considering all relationships between adjacent months, all measured in their trading horizon, leads to the monthly weighting factors as displayed in figure 3.
Shape shifting
The monthly price forward curve can be derived separately for baseload and peakload but, ultimately, market participants require an hourly-shaped curve. Hourly shapes can undergo structural changes over time. For example, Germany’s increase in solar power production over the past decade – to a level where solar can now sometimes fulfil more than 50% of total demand – has caused the hourly shape to gradually, but steadily flatten, turning from a pyramid into a camel (see figure 4).
Capture rates
To understand how the forward curve influences the value of a PPA contract, a capture rate is used. A high average (expected) power price and a high baseload level of the forward curve, increases the expected income of the asset. But this is not the only relevant factor. For an individual asset, it is the capture price that counts. This is the weighted average price at which the asset produces. The capture rate is this capture price divided by the baseload price. A power plant producing baseload has a capture rate of 100%. A peaking unit, or flexible hydro power plant only produces when power prices are high, and hence has a capture rate of, for example, 200% (but with much lower total production). Non-dispatchable renewable generation assets mostly have a capture rate below 100%.
This is the so-called cannibalisation effect: with more and more production from the same type of renewable generation, the realised (capture) prices go down. When an individual wind turbine or solar panel produces power, many others do so too, so the average capture price is low, sometimes even dropping to zero or negative.
Long-term forecasting
While exchanges are gradually extending the horizon of futures trading – EEX, for example, goes out six years and may soon stretch to 10 years – beyond three to five years in most markets there is virtually no liquidity or transparency and therefore no good market benchmark for the power price.
Renewable energy investments have an economic lifetime of at least 15 years and hence investors seek long-term PPA contracts to provide financial security. Both PPA buyer and seller have to form an opinion about the longer-term level of power prices. It is impossible to forecast the future power price over such horizons with high accuracy, but it is possible to form a view using a fundamental power market model. The further into the future the assessment, the more fundamental changes are going to affect the power prices.
Beyond the merit order
Fundamental analysis, also named the ‘merit-order approach’ provides a future baseload price forecast, but also the shaping cost or capture rate. The basic idea is that the power price is a result of supply and demand. In a competitive market, each plant owner bids its supply into the market at a price that makes it just profitable to produce. At any given hour, the plants with the lowest bids produce the needed power. The last plant that is required to fulfil demand, is the price-setting plant in the merit order.
It is not trivial to determine which plant will be the price-setting plant. For example, it is important to take into account start costs, various plant operational constraints, energy storage and interconnection capacities to name a few of the many important features of real-life power markets. A simple merit order based on average production costs is a useful starting point, but lacks sufficient detail for any serious investment analysis or PPA valuation.
Multiple inputs
A more accurate fundamental analysis will cover many power market areas, taking in multiple inputs. It’s necessary to work out the residual load – the forecast of power demand, minus the forecast of non-flexible generation from solar, wind and hydro. Fuel and carbon price forward curves and detailed information about power generation assets need to be calculated in order to determine the variable production costs of all flexible generation assets. Batteries and other forms of energy storage also need to be included in the power plant list. Finally, it’s necessary to consider interconnection capacities that allow power to flow from low-priced areas to high-priced areas until prices are equal in both areas or until the capacity is fully utilised.
Using all of those inputs, the fundamental model finds out exactly which plant will be producing at which hour and at what costs. It provides a wealth of information, including the total fuel consumption, carbon emissions and interconnection flows. It also results in gross margin estimates for each individual plant and energy storage, and the conditions under which the market is undersupplied or oversupplied.
Fundamental models need to make assumptions about future supply and demand levels. Figure 5 shows annual forecasts for six European power markets, with a gradually rising price trend, especially after 2025. The fuel forward prices, determining the costs of conventional generation, are taken from settlement prices on April 14, 2020 and extrapolated by 2% annually after 2025. The carbon prices are from the same trading date, and extrapolated a bit more aggressively by 5% annually after 2027.
Among the many fundamental modelling assumptions for this are a small increase in electricity consumption and a strong rise in solar and wind capacities. These increases are projected on the actual demand, solar production and wind production in 2018, as if the future weather conditions follow the 2018 weather conditions exactly.
In this context, 2018 is the so-called base year for the residual load forecast, making the results more realistic than with an averaged residual load forecast. In order to generalise the results, the model can be run with multiple base years, for example 2016 and 2017.
The fundamentally generated price forecasts can be compared to forward market prices. Figure 6 shows forecasts for German power prices compared with EEX settlement prices from 2021–26. EEX prices are below the fundamental forecast by €2/MWh on average in 2021–23, and above the fundamental forecast by €1.3/MWh in 2024–26. A potential explanation for the first three years is that the Covid-19 effect was not taken into account in the fundamental model, whereas the market might have priced in the effects of a demand slump, especially in 2021.
Fundamental analysis also calculates hourly price levels. Figure 7 shows these in a two-month period in 2031. On the same day, prices might vary from below €10 to above €70/MWh.
Avoiding blackouts
Over the years, there will be changes in the conventional generation park. Some of these changes can be derived directly from government plans and commercial investment and de-investment announcements. For example, nuclear capacities are to be phased out in Germany by 2023 and in Belgium by 2025. In Germany, this coincides with a gradual phasing out of coal and lignite plants by 2035–40. Because net electricity demand is not expected to decrease, and because solar and wind are not always available, the maximum required capacity remains roughly the same. Consequently, the system would have blackouts in certain periods if this decrease in flexible generation assets were not compensated for by an increase in energy storage.
So, most of the assumed increase in gas-fired generation and energy storage is based on the prerequisite that society will not accept blackouts. Some of these new capacities will earn enough money in the day-ahead power markets, while others cannot do without the income from ancillary services to balance the system. And, in some cases, even capacity fees might be needed for a sound economic business case.
Valcoe, not LCOE
It has become standard practice to compare the economics of renewable power technologies by their levelised cost of energy (LCOE). The LCOE equals the fixed and variable costs over the lifetime of the asset, divided by the number of production hours. However, this concept does not take into account the level of the power price in the hours of production. It also ignores the flexibility that some assets have to provide balancing services and it ignores the cannibalisation effect.
An improved concept introduced by the International Energy Agency is the value-adjusted LCOE, or Valcoe. For intermittent sources, it adds the cannibalisation effect and balancing costs to the variable costs. Likewise, for flexible sources, it reduces the costs by the expected income from ancillary services. The net result is a fairer economic comparison between technologies providing flexibility (energy storage, most conventional assets) and requiring flexibility (most renewable assets).
Forecasting capture rates
The Valcoe highlights the importance of the capture rate in the economics of a renewable asset. So far, capture rates have been fairly close to 100%. With increasing levels of renewables penetration, capture rates are likely to be pushed down. This has important implications for the value of long-term PPAs and the negotiated terms.
Calculating future capture rates, as well as prices, is therefore essential, and fundamental analysis is key. Of course, many assumptions have to be made and, in practice, this means running several scenarios and calculating sensitivities to deepen the understanding of an asset or PPA in order to be prepared for any price negotiation.
Cyriel de Jong is founder and director at Kyos Energy Analytics. Read the first article in this renewable power series here.
Only users who have a paid subscription or are part of a corporate subscription are able to print or copy content.
To access these options, along with all other subscription benefits, please contact info@risk.net or view our subscription options here: http://subscriptions.risk.net/subscribe
You are currently unable to print this content. Please contact info@risk.net to find out more.
You are currently unable to copy this content. Please contact info@risk.net to find out more.
Copyright Infopro Digital Limited. All rights reserved.
You may share this content using our article tools. Printing this content is for the sole use of the Authorised User (named subscriber), as outlined in our terms and conditions - https://www.infopro-insight.com/terms-conditions/insight-subscriptions/
If you would like to purchase additional rights please email info@risk.net
Copyright Infopro Digital Limited. All rights reserved.
You may share this content using our article tools. Copying this content is for the sole use of the Authorised User (named subscriber), as outlined in our terms and conditions - https://www.infopro-insight.com/terms-conditions/insight-subscriptions/
If you would like to purchase additional rights please email info@risk.net
More on Commodities
Energy Risk 2024 Software Rankings: IT demands increase amid rising risk
Heightened geopolitical and credit risk increase requirements on commodities software
Energy Risk Asia Awards 2023: the winners
Winning firms demonstrate resiliency and robust risk management amid testing times
ION Commodities: addressing the market’s recent pain points
Energy Risk Software Rankings winner’s interview: ION Commodities
Energy Risk Commodity Rankings 2023: adapting to new market dynamics
Winners of the 2023 Commodity Rankings provided reliability when clients faced extreme change
Energy Risk Software Rankings 2023: managing uncertainty
Unpredictable markets make CTRM software choices key
Navigating the volatility and complexity of commodity markets
Commodity markets have experienced significant challenges since the Covid-19 pandemic, the conflict in Ukraine and the subsequent sanctions imposed on Russia. These unprecedented events have caused fluctuations in supply and demand, disrupted global…
Energy Risk Asia Awards 2022
Recognising excellence in energy risk management
Market shrugs off EC’s plan to change gas benchmark
Dutch TTF prices unmoved, as market participants say they are “not taking it seriously”